Solar Energy - A Strategic Response to Power Outages
Providing the entire US energy requirements of 28,000 billion kWh per year would thus require a total collecting area of 17.5 million ha. That is less than the area presently occupied by hydroelectric power plants.
We don't know the exact cause of the August 14th US/Canadian power outage, but it was an obvious demonstration of both countries' vulnerability to loss of power. It's also known that the area was under an extreme heat wave and even at 4 PM, the solar energy resource was nearly ideal as shown by the GOES satellite picture. The next morning's newscasts stated that alternatives to our current power system are much too costly…but are the alternatives that costly? What about solar and efficient use of energy?
First, let's consider some common myths about solar energy. The greatest of these myths being the amount of land required for photovoltaics, or solar electric panels commonly called PV.
- A conservative estimate of the solar energy reaching the ground in the United States is 1,500 kWh per square meter per year (actually ranging from 1200 to over 2000).
- With 15% solar-to-electricity conversion via PV[a], usable energy is 1500x0.15 = 225 kWh/sq.m/yr[b].
- Assuming that hydrogen would be used as the primary energy storage medium, and using 70% for energy-storage-energy round trip efficiency via, e.g., fuel cells, the useable energy collectable by unit of ground surface is 225x0.7 = 158 kWh/sq.m/yr.
Providing the entire US energy requirements of 28,000 billion kWh per year would thus require a total collecting area of 17.5 million ha. That is less than the area presently occupied by hydroelectric power plants.
Because solar technologies such as PV are highly modular and can be incorporated in common building materials such as glass and roofing products, much of this resource could be deployed on already urbanized landscapes, near points of the greatest electricity use. It would only take a small percentage ~ 15% of urbanized land -- consisting of buildings, highways, parking lots, exclusion zones, to build the PV power structures.
But, back to the question of the cost of such a huge deployment of solar generation capability. It will, no doubt, be staggering. But, as a society, we could certainly afford it: let's just put this affirmation in perspective by considering the following scenario.
What if, starting in 1973 (the oil embargo year), we had invested the amount we spend yearly on fossil fuel subsidies -- $40 billion - in deploying PV power plants at market price?
Noting that back in 1973, $40 billion in current dollars was worth ~ $10 billion and PV installations cost was $35/Watt; assuming that massive and consistent purchases would have induced yearly cost reductions of 5% per year, down to a minimum achievable cost of $ 1.50/Watt; further assuming a 10% overhead on initial investments, reinvestment of 70% of systems' revenues in new PV systems (breeder effect), systems' output degradation of 1% a year, and systems' maximum life span of 30 years, the total installed PV capacity in the US today (2003) would be equal to 64 GW and growing at an exponentially accelerating rate of 8 GW per year.
Continuing under these assumptions, PV would be in a position to provide 100% of the US energy consumption by 2044….
However, the investment was not made, and a truly robust energy infrastructure is only achieved with both diversity of fuel resources and technologies. Investments should be made in all resources with a plan to decrease fossil fuel dependency to a level of equal to the US production and increase investments in both renewable technologies such as wind, solar, biomass, while making sure that future investments in the electricity grid incorporate design features amenable to renewable technologies.
Solar energy, placed on customer sites and used where it's needed, becomes one strategic option to prevent total grid shut down.
Featured Product
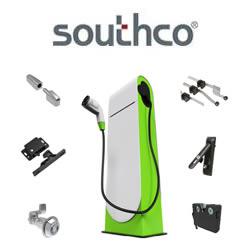