Although weaning off fossil fuels in favor of nuclear fusion will take massive amounts of time and money, this incredible feat for man will be a giant leap for all of mankind.
Nuclear Fusion : Could This Be the Next Thing?
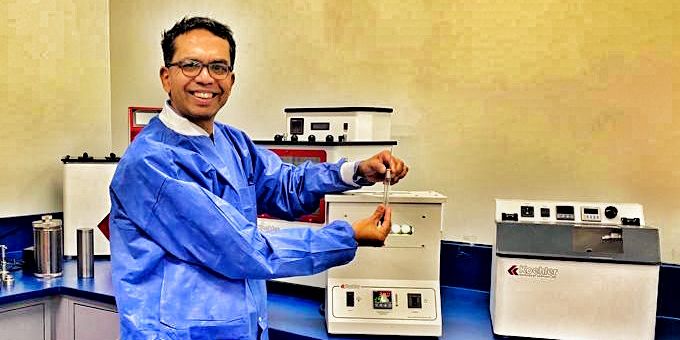
Dr. Raj Shah and William Streiber | Koehler Instrument Company
December 5th, 2022 will be marked as one of the landmarks on the sustainability of nuclear fusion timeline. Researchers at the Lawrence Livermore National Laboratory in California successfully executed a nuclear fusion reaction that resulted in a net energy gain [1]. Of the 2.05 megajoules of energy used to power the world’s largest laser at the National Ignition Facility (NIF), 3.15 megajoules were harvested yielding a net gain of 1.10 megajoules [1]. This energy is sufficient to boil approximately 10 kettles of tea water [2]. Though infinitesimal in comparison to the energy consumed by the NIF, this net gain opens the door for a clean, sustainable, alternative to fossil fuels. Although weaning off fossil fuels in favor of nuclear fusion will take massive amounts of time and money, this incredible feat for man will be a giant leap for all of mankind.
Nuclear fusion is defined as the reaction in which atomic nuclei of low atomic number fuse together to form one heavier nucleus, along with the release of energy [3]. This process creates the energy of the sun, where hydrogen isotopes deuterium and tritium, fuse to form helium atoms and a large amount of energy. Renowned for its production of mass amounts of energy, researchers have been attempting to produce controlled nuclear fusion for almost half a century using a process known as inertial confinement fusion (ICF) [4]. ICF uses a tiny amount of solid fuel, usually deuterium-tritium (D-T), contained in a small pellet. This fuel is then compressed to tremendous density and temperature [4]. This compression is necessary to produce fusion power before the fuel pellet is blown apart. The desired density and temperature are achieved by using an intense laser beam, which is referred to as the driver [4]. In an ICF reaction, there are two distinct types of drivers, indirect and direct drive. In direct drive ICF, the capsule or fuel pellet relies of electron conduction for energy transport to drive the implosion [5]. Simply, in a direct drive ICF process, the laser is aimed directly at the target [5]. Conversely, indirect drive relies on x rays for energy transport. The energy from the laser is first absorbed in a high-Z enclosure, known as a hohlraum [5]. This hohlraum is used to smooth the distribution of radiation seen by a fuel-filled capsule [6]. In other words, a uniform X-ray radiation bath is created by the hohlraums, which then motivates the fuel filled capsule to implode [5],[6]. The NIF uses the indirect drive ICF process. For fusion to be a viable source of sustainable electricity, two physical conditions must be met. The first is that the temperature be in the region of 20 kiloelectronvolts (keV) [7]. Secondly, the hot plasma (i.e., the D-T fuel), should be adequately confined; meaning the product of the number density and the confinement time should lie in the range of 10^15 seconds/cm^3 [7]. This condition corresponds to the idea of Lawson criterion and the two conditions simply ensure that a sufficient number of interactions occur, and the fuel will be consumed [7].
In the field of fusion reactions, there are two main subdivisions. The first is the previously discussed inertial confinement fusion (ICF) and the other is magnetic confinement fusion (MCF). In MCF, a giant, powerful, magnetic field is employed to confine the movement of deuterium-tritium plasma [8]. However, this method, especially when first used in the 1940s, was found to be highly unstable due to a lack of sufficient energy confinement methods [8]. This instability has resulted in a greater focus on ICF reactions, and the breakthrough seen this past year.
This ICF reaction differed from others before it in multiple ways, causing the gain in energy observed in the “record shot”. One factor was that the power of the laser was increased by 10%. This increase occurred because while the laser always had the ability to increase its power, out of an abundance of caution this power was never reached for the previous power of the laser was deemed appropriate. Additionally, great strides have been made recently in the hardening of the optics, and researchers felt it safe to increase the power of the laser (all physics today cite). The stronger the laser, the more intense the light produced by the laser which in turn heightens the intensity of the X-rays emitted by the hohlraum. This assisted in the ignition process of the capsule, increasing the efficiency of the reaction, and enabling the newly acquired net energy gain.
Additionally, thickness of the D-T fuel pellet (or capsule) improved the ignition and fusion process. There are two distinct types of shell coatings used in ICF reactions, single shell, and double shell capsules. It has been found that double-shell capsules, which contain a high-Z inner shell and a low-Z ablator, or outer shell, achieve ignition at lower peak ignition speeds and do not require precise shock sequencing [9]. This stability in kinematics is traded in for increased mixing of the fuel and shell, decreasing the performance of ignition due to depressed purity of fuel [9]. The NIF utilizes single shelled, nanocrystalline diamond coated capsules containing ignition fuel [10, 11]. In the record shot, a diamond shell about 10% thicker than was previously used was instituted [11]. These diamond shells, upon blasting with X-rays via the hohlraum, blow off like a rocket, creating the implosion [11]. Upon further study of the capsules, it was realized that major defects were initially unaccounted for in the experimental calculations. Most influential was the unknown inflated mixing of the capsule material and the D-T fuel. This mixing led to negative performance of implosions, as the D-T fuel had lessened purity. For the fusion reaction to succeed, the capsule needs to be compressed by 30-45x [12]. This means that any nonuniform distribution of X-rays will have an amplified effect on the ignition. It is this uneven blasting of the capsule with X-rays that cause the mixing of the capsule shell and the ignition fuel. This is due to hydrodynamic instabilities and leads to cold ablator material integrating with the heated fuel [13]. Improving the thickness of the capsule decreases the effect of these hydrodynamic instabilities for the thicker shell gives the capsule higher hydrodynamic resistance [14]. This resistance forces the capsule to absorb additional X-rays to cause implosion. This heightened resistance necessitates greater uniformity in energy distribution compared to the thinner shell for more energy will be needed to cause the ignition, giving the capsule more time to achieve uniform energy distribution. Therefore, the thicker shell reduces the mixing of the fuel and capsule shell and permits improved purity of fuel for ignition. This improved purity is a major distinction between the record shot and fusion reactions before it. This enhanced purity of the D-T fuel caused by the improved hydrodynamic resistance of the thicker shell is one factor responsible for the net energy gain seen in the latest ICF reaction at the NIF.
In this breakthrough reaction, an indirect drive ICF reaction was used. The amount of energy placed into the high beam driver, 2.05 mega joules of energy, amounted in a harvested energy after implosion of 3.05 megajoules [1]. This net energy gain as previously stated is unprecedented and has never been achieved until now. While this net energy gain is a phenomenal accomplishment, reliance on fossil fuels will remain the backbone of energy supply for the foreseeable future. The 1.05 megajoules produced from the reaction is just 1% of the 300 megajoules form the grid which powers the 192 laser National Ignition Facility [11]. Figure 1 below shows how minuscule this energy gain is in comparison to the energy expended from the grid to produce it.
Figure 1 – Energy Produced versus Energy Expended
Reprinted from [11]
Additionally, the NIF at Lawrence Livermore National Laboratory cost 3.5 billion USD to build, and the average energy cost of running an ICF reaction is approximately 130 times the energy produced [15]. These two bureaucratic hurdles, along with engineering hurdles, such as mass production of fusion capsules and the firing of lasers continually lead researchers and skeptics to believe that this breakthrough is overexaggerated.
This skepticism, as well as the monetary and manufacturing hurdles that nuclear fusion currently faces, support the idea that nuclear fusion will not be viable as a sufficient energy source for decades. However, this revolutionary finding brings into effect a reinvigorated enthusiasm which may be the spark that brings nuclear fusion back into the playing field of energy production. Offering a clean, reliable source of energy, nuclear fusion will be a heavily researched field in the years to come, despite its obstacles and hurdles. In all, this finding will help rekindle interest in the field of nuclear fusion energy both academically and monetarily. Both interests, spent wisely, will likely bring about long term change necessary to jump these manufacturing hurdles and bring nuclear fusion to the table as a viable means for energy production and possible energy dependence in the United States.
About Dr. Raj Shah
Dr. Raj Shah is a Director at Koehler Instrument Company in New York, where he has worked for the last 27 years. He is an elected Fellow by his peers at IChemE, CMI, STLE, AIC, NLGI, INSTMC, Institute of Physics, The Energy Institute and The Royal Society of Chemistry. An ASTM Eagle award recipient, Dr. Shah recently coedited the bestseller, “Fuels and Lubricants handbook”, details of which are available at ASTM’s Long-Awaited Fuels and Lubricants Handbook 2nd Edition Now Available (https://bit.ly/3u2e6GY). He earned his doctorate in Chemical Engineering from The Pennsylvania State University and is a Fellow from The Chartered Management Institute, London. Dr. Shah is also a Chartered Scientist with the Science Council, a Chartered Petroleum Engineer with the Energy Institute and a Chartered Engineer with the Engineering council, UK. Dr. Shah was recently granted the honourific of “Eminent engineer” with Tau beta Pi, the largest engineering society in the USA. He is on the Advisory board of directors at Farmingdale university (Mechanical Technology ) , Auburn Univ ( Tribology ), SUNY, Farmingdale, (Engineering Management) and State university of NY, Stony Brook ( Chemical engineering/ Material Science and engineering). An Adjunct Professor at the State University of New York, Stony Brook, in the Department of Material Science and Chemical engineering, Raj also has over 550 publications and has been active in the energy industry for over 3 decades. More information on Raj can be found at https://bit.ly/3QvfaLX
About William Streiber
William Streiber is part of a thriving internship program at Koehler Instrument company and a student of chemical engineering at State University of New York, Stony Brook, where Dr. Shah currently heads the External advisory board of directors.
References
-
Clifford, C. (2022, December 14). Fusion power is still decades and billions of dollars away, even after this week's major scientific breakthrough. CNBC. Retrieved January 7, 2023, from https://www.cnbc.com/2022/12/14/fusion-power-wont-be-widespread-for-at-least a-decade.html
-
Nilsen, E., & Marsh, R. (2022, December 13). US scientists reach long-awaited nuclear fusion breakthrough, source says | CNN politics. CNN. Retrieved January 7, 2023, from https://www.cnn.com/2022/12/12/politics/nuclear-fusion-energy-us-scientists-climate/index.html
-
Barbarino, M. (2022, March 31). What is nuclear fusion? IAEA. Retrieved January 7, 2023, from https://www.iaea.org/newscenter/news/what-is-nuclear-fusion
-
Prager, S. C., & Najmabadi, F. (2022, September 14). Mirror confinement. Encyclopædia Britannica. Retrieved January 7, 2023, from https://www.britannica.com/technology/fusion-reactor/Mirror-confinement
-
Lindl, J. (1995, November 1). Development of the indirect‐drive approach to Inertial Confinement Fusion and the target physics basis for ignition and gain. AIP Publishing. Retrieved January 7, 2023, from https://aip.scitation.org/doi/10.1063/1.871025
-
Li, X., Wu, C., Dai, Z., Zheng, W., Zhao, Y., Zhang, H., Gu, J., Kang, D., Ge, F., Gu, P., & Zou, S. (n.d.). REVIEWOFTHETHREECANDIDATEHOHLRAUMSINICF - arxiv.org. Retrieved January 8, 2023, from https://arxiv.org/pdf/1608.02167.pdf
-
Keefe, D. (n.d.). Inertial confinement fusion - annual reviews. Inertial Confinement Fusion. Retrieved January 8, 2023, from https://www.annualreviews.org/doi/10.1146/annurev.ns.32.120182.002135
-
McCracken, G., & Stott, P. (2007, September 2). Magnetic confinement. Fusion. Retrieved January 7, 2023, from https://www.sciencedirect.com/science/article/pii/B978012481851450007X
-
Gunderson, M., Daughton, W., Simakov, A., Wilson, D., Watt, R., Delamater, N., & Montgomery, D. (2015, November). Implosion dynamics and mix in double-shell ICF capsule designs. NASA/ADS. Retrieved January 9, 2023, from https://ui.adsabs.harvard.edu/abs/2015APS..DPPTP2027G/abstract
- Montgomery, D. S., Daughton, W. S., Albright, B. J., Simakov, A. N., Wilson, D. C., Dodd, E. S., Kirkpatrick, R. C., Watt, R. G., Gunderson, M. A., Loomis, E. N., Merritt, E. C., Cardenas, T., Amendt, P., Milovich, J. L., Robey, H. F., Tipton, R. E., & Rosen, M. D. (2018, September 27). Design considerations for indirectly driven double shell capsules. Physics of Plasmas. Retrieved January 9, 2023, from https://www.osti.gov/pages/servlets/purl/1477699
- Kramer, D. (2022, December 13). National Ignition Facility surpasses long-awaited fusion milestone. Physics Today. Retrieved January 9, 2023, from https://physicstoday.scitation.org/do/10.1063/PT.6.2.20221213a/full/
- Weber, C. R., Casey, D. T., Clark, D. S., Hammel, B. A., MacPhee, A., Milovich, J., Martinez, D., Robey, H. F., Smalyuk, V. A., Stadermann, M., Amendt, P., Bhandarkar, S., Chang, B., Choate, C., Crippen, J., Felker, S. J., Field, J. E., Haan, S. W., Johnson, S., … Sepke, S. M. (1970, January 1). Improving ICF implosion performance with alternative capsule supports. AIP Publishing. Retrieved January 9, 2023, from https://aip.scitation.org/doi/10.1063/1.4977536
- D E Hinkel et al 2013 Plasma Phys. Control. Fusion 55 124015. IOP Publishing. Retrieved January 9, 2023, from https://iopscience.iop.org/article/10.1088/0741 -3335/55/12/124015/pdf
- Sajeesh, P., Doble, M., & Sen, A. K. (2014, October 6). Hydrodynamic resistance and mobility of deformable objects in microfluidic channels. Biomicrofluidics. Retrieved January 9, 2023, from https://www.ncbi.nlm.nih.gov/pmc/articles/PMC4222326/
- Hartsfield, T. (2022, December 14). There is no "breakthrough": NIF Fusion Power still consumes 130 times more energy than it creates. Big Think. Retrieved January 9, 2023, from https://bigthink.com/the-future/fusion-power-nif-hype-lose-energy/
The content & opinions in this article are the author’s and do not necessarily represent the views of AltEnergyMag
Comments (0)
This post does not have any comments. Be the first to leave a comment below.
Featured Product
