Bioethanol is a potential alternative energy source that, through investment into infrastructure and its related industries, can lead the way in reducing emissions. The purpose of this article is to highlight bioethanol’s advancements and its future in various industries.
Bioethanol : Recent advances & potential
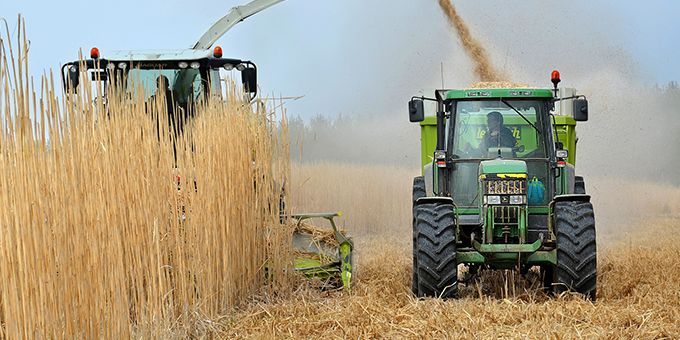
Dr. Raj Shah, Nicholas Douglas and Nathan Aragon | Koehler Instrument Company
Due to a rise in global temperatures, many fuel industries have searched for alternative energy sources that lower emissions rates in combustion engines. Although new energy sources show promise in reducing emissions and costs, large investments and arduous construction of infrastructure must occur for any effect to take hold. Bioethanol is a potential alternative energy source that, through investment into infrastructure and its related industries, can lead the way in reducing emissions. The purpose of this article is to highlight bioethanol’s advancements in various industries and its future in those industries.
Bioethanol has many applications in several industries across the world. Applications include fuel for power in combustion processes, fuel for fuel cells, feedstock for chemical companies, and fuel for cogeneration systems [1]. The broad application of bioethanol benefits current engines and fuel processes, serving as an alternative to regular petrol in engines. As an engine fuel, bioethanol can serve as an alternative for gasoline at any blend percentage, creating more efficient engines in the process [1]. Current engines tolerate up to 15% bioethanol blends without modifications. Bioethanol reduces emissions due to how it is produced [1]. One method of production involves using lignocellulose to break down biomass, and then extracting its sugars through using enzymes and hydrolysis [1].
As an alternative energy source, bioethanol helps reduce emissions. Since much of bioethanol comes from seed cultivation and harvesting, emissions have a much lower carbon intensity compared to other fuels [2]. Figure 1 shows the carbon intensity of various bioethanol and biodiesel emissions. Bioethanol emissions have a much lower carbon intensity due to lower indirect land use exchange (iLUC). Otherwise, the direct emissions of bioethanol fuels range from 15 to 65 grams of CO2 emissions per megajoule (gCO2e/MJ) [2]. Although direct emissions from bioethanol and biodiesel compare in values, total bioethanol emissions remain low due to low iLUC. Also, European bioethanol emission trends between 2009 and 2013 show total greenhouse gas (GHG) emissions decrease from 42.9 gCO2e/MJ to 33.9 gCO2/MJ [2]. Continued downward trends in emissions will benefit widespread bioethanol use in the future.
Advancements in production and applications have made bioethanol a promising alternative to fossil fuels. Researchers have looked at ways to improve current processes. This included analyzing which raw materials were best. As a renewable resource, bioethanol comes from various plants and fungi, such as willow, spruce, and different cereals [3]. Researchers found that prime bioethanol production would be in tropical climates due to nutrient-rich soils that reinforce plants. Also, it was found that Miscanthus giganteus (elephant grass) and Trichoderma reesei exhibit properties beneficial to bioethanol fuels. For the Miscanthus plant, it contains a high calorific value at approximately 4200 kcal/kg, which would drastically improve any fuel combustion. For the Trichoderma fungus, it breaks down the lignocellulose bonds found in raw materials used for bioethanol production [3]. Investment into farms for Miscanthus plants and increased use of Trichoderma fungi to assist in bioethanol production would increase production and make bioethanol a viable fuel in several industries.
Figure 1: Carbon intensity of various bioethanol and biodiesel fuels [2]
Various studies have been performed to determine which plants produce the optimal bioethanol fuel. In a study of various Miscanthus genomes on bioethanol production, researchers found that the Giganteus genome, which was previously mentioned for its beneficial properties in bioethanol production, had the lowest yield rate at 185-222 g/kg DM [4]. Among three Miscanthus genomes researchers analyzed, the Sinensis (Chinese silver grass) genome had the highest yield at 234-253 g/kg DM, making it viable for efficient bioethanol production. Also, all crops were grown in similar conditions in west-central Poland, and researchers observed the yield rates of these crops as they were cultivated in a three-year period [4]. M. sinensis production may be increased to promote efficient bioethanol fuel in many industries due to its high bioethanol yield.
Research supports bioethanol as a fuel due to its efficient performance in engines and low emission rate from combustion. Researchers investigating the effects of bioethanol on spark-ignition (SI) engine performance and emissions found bioethanol having great benefits to overall fuel performance and emission reductions. For fuel performance, bioethanol fuel outperformed gasoline in engine speed and brake specific fuel consumption (BSFC) when testing for the effects of air temperatures and engine speeds [5]. Figures 2a and 2b show the effect of air intake on both volumetric capacity (%) and BSFC (g/kWh). For volumetric capacity, a lower air temperature produced a more efficient bioethanol than gasoline, with the best results at 10o C [5]. However, as engine speed increased, volumetric capacity decreased for both gasoline and bioethanol. Also, bioethanol’s BSFC showed significant improvements over gasoline, with results at all three air temperatures between 400 and 425 g/kWh [5]. The enhanced performance of engines using bioethanol may lead to widespread usage, pending investment and efficient production.
Figure 2a: Effects of bioethanol and gasoline on volumetric capacity (%) and engine speed (rpm) [5]
Figure 2b: Effects of bioethanol and gasoline on BSFC (g/kWh) and engine speed (rpm) [5]
Although bioethanol fuel promises enhanced engine performance in SI engines, challenges during engine performance must be researched and overcome for economic viability. One particular challenge is its high boiling point of 78o C, which leads to cold start problems [5]. Consumers who use bioethanol would have difficulties driving in colder temperatures, potentially losing fuel while it heats to the boiling point. One possible solution could involve mixing bioethanol with gasoline or other additives to lower the boiling point to suit colder temperatures.
Within bioethanol production, several advancements have been made to increase its efficiency as a fuel. Current processes include hydrolysis & fermentation, and simultaneous saccharification and fermentation. Researchers have looked to improve on such processes to make bioethanol an economically and environmentally stable fuel. Researchers have investigated bioreactor designs and biorefineries to drastically improve the commercial success of bioethanol products. As for bioreactors, two types - external loop liquid lift and circulating loop bioreactors - were under development for bioethanol production. External loop liquid-lift bioreactors were utilized for simultaneously running mass transfer and fermentation of crop biomass [6]. This would drastically enhance the bioethanol fuel, reducing costs and creating a more efficient fuel for use. Circulating loop bioreactors served as modifications to external loop air-lift bioreactors, created for both anaerobic and aerobic processes to happen in the same space [6]. Also, direct ethanol extraction occurred using raw cassava starch. While there were plants for single-step production of bioethanol, researchers designed and developed these reactors to deal with complex processes, such as co-fermentation [6]. Figure 3 below shows the typical “energy-driven” biorefinery, used to produce a variety of products.
Figure 3: “Energy-driven” biorefinery and its processes [7]
Hydrolysis and fermentation, while important processes in bioethanol production, have challenges that researchers must investigate to create a more efficient process. In a study on membrane processes in the bioethanol industry, researchers found that separate hydrolysis and fermentation (SHF) can be optimized to efficiently produce bioethanol [8]. Since both processes can be optimized separately, producers can change how much bioethanol is produced and at what quality [8]. The challenge facing researchers is how to promote SHF over simultaneous hydrolysis and fermentation (SFF). SFF is more abundant because both processes involve a single step, most likely decreasing operational costs for producers. However, by investing in SHF processes, bioethanol production through membranes can greatly benefit several companies. Another challenge, particularly with fermentation, is which method of loading is best for fermentation. In a study of sugar beet pulp for bioethanol production, researchers found that high-solid loading, which involves large amounts of biomass, has low recovery rates and sugar inhibition [9]. To overcome this problem, researchers used simultaneous saccharification and fermentation (SSF) to create bioethanol fuel, leading to efficient results [9]. This includes higher bioethanol concentrations while under fed-batch fermentation by up to 6 mg/mL more bioethanol [9].
As for the future, research shows that bioethanol has great potential for its respective industries. Many industries use bioethanol to create certain products, including the energy, chemical, and food industries. In the energy industry, various engines and industrial equipment use bioethanol as fuel. China New Energy, a Chinese fuel company specializing in biofuels, leads in bioethanol production and exports [10]. Bioethanol produced from China New Energy is used as fuel, chemical solvents, and even beverage ingredients, as edible bioethanol grows in popularity [10]. China New Energy also patented 22 advanced bioethanol production technologies, including advanced fermentation and distillation equipment [10]. Another bioethanol company, Greenbelt Resources Corporation, produces bioethanol as a fuel from food waste, paving the way for eco-friendly bioethanol production [10].
The potential of bioethanol lies in the genetic engineering of crops, which could greatly increase biomass output and make deconstruction of lignocellulose easier [11]. Since more biomass can lead to more ethanol, a quicker deconstruction of lignocellulose to its basic starches would decrease manufacturing costs all around. Research has also been performed on bioethanol production from food crops. However, researchers have found this option undesirable because bioethanol production would take away from food supply. As the human population increases, more food is required to sustain healthy populations. Therefore, interference in the food supply would detriment several countries in desperate need of those crops, forcing researchers to find a way to efficiently deconstruct lignocellulose [11].
Bioethanol production from lignocellulose is an economically and environmentally friendly method of production. As mentioned before, bioethanol research points to lignocellulose-based production as a reality due to little impact on food supply. In a study on bioethanol production from straw, it was found that the conversion from lignocellulose can create major economic incentives due to its abundance in various straws and low cellulosic costs [12]. Although promising, many obstacles lie in the way of researchers creating the perfect lignocellulosic bioethanol production process; this includes producing a method easily deconstructing lignocellulose in an efficient manner [12]. Biorefineries, once readily available, would assist in placing bioethanol on a large market scale.
Researchers have found that lignocellulosic sources such as hardwoods, softwoods, waste paper, and municipal solid wastes can all efficiently create bioethanol [13]. The lignocellulosic biomass is then pretreated to break down the biomass into cellulose and other polymers, which then undergo hydrolysis and fermentation processes. To create an economically viable process, optimization of sugar recovery after pretreatment, xylose fermentation by yeast, and lower enzyme & yeast loading must occur [13]. Since filtration would be excluded due to whole pretreated material included, operation costs would decrease. However, more efficient microorganisms must be developed to determine how much the lignocellulosic bioethanol industry grows [13].
In conclusion, bioethanol promises great benefits as an alternative to current fuels because of its economic potential, lower emissions, and higher fuel efficiency. Indeed, bioethanol’s vast applications in several industries appeal to companies looking for a safer alternative to already existing fuels. Investment into lignocellulosic bioethanol research and infrastructure can create new opportunities to lower emissions and create a greener environment.
The authors are grateful to Mr. David Forester for his review of this manuscript.
Authors
Dr. Raj Shah is a Director at Koehler Instrument Company in New York, where he has worked for the last 25 years. He is an elected Fellow by his peers at IChemE, CMI, STLE, AIC, NLGI, INSTMC, The Energy Institute and The Royal Society of Chemistry. An ASTM Eagle award recipient, Dr. Shah recently coedited the bestseller, “Fuels and Lubricants handbook”, details of which are available at
https://www.astm.org/DIGITAL_LIBRARY/MNL/SOURCE_PAGES/MNL37-2ND_foreword.pdf
A Ph.d in Chemical Engineering from The Penn State University and a Fellow from The Chartered Management Institute, London, Dr. Shah is considered an expert in alternative energy technologies. He is also a Chartered Scientist with the Science Council, a Chartered Petroleum Engineer with the Energy Institute and a Chartered Engineer with the Engineering council, UK. An adjunct professor at the Dept. of Material Science and Chemical Engineering at State University of New York, Stony Brook, Raj has over 300 publications and has been active in the petroleum and alternative energy fields for the past 3 decades. More information on Raj can be found at
Mr. Nicholas Douglas and Mr. Nathan Aragon are students of Chemical engineering at SUNY, Stony Brook University, where Dr. Shah is an adjunct professor and the chair of the external advisory Committee in the Dept. of Material Science and Chemical Engineering.
References
[1] Pirolini, Alessandro. “What Is Bioethanol?” AZoCleantech.com, AZoCleantech , 4 June 2015, www.azocleantech.com/article.aspx?ArticleID=403.
[2] Szabo, Zoltan. “Reviewing the GHG Savings of Ethanol - WebDream.” Eerl.com, EthanolEurope, Nov. 2014, www.eerl.com/Uploads/Reviewing-the-GHG-savings-of-Ethanol-Nov-2014.pdf.
[3] Di Nicola, Giovanni, et al. “Advances in the Development of Bioethanol: A Review.” Biofuel's Engineering Process Technology, 1 Aug. 2011, doi:10.5772/22510.
[4] Cerazy-Waliszewska, Joanna, et al. “Potential of Bioethanol Production from Biomass of Various Miscanthus Genotypes Cultivated in Three-Year Plantations in West-Central Poland.” Industrial Crops and Products, vol. 141, 25 Sept. 2019, p. 111790., doi:10.1016/j.indcrop.2019.111790.
[5]Yoon, Seung Hyun, and Chang Sik Lee. “Effect of Undiluted Bioethanol on Combustion and Emissions Reduction in a SI Engine at Various Charge Air Conditions.” Fuel, vol. 97, 15 Feb. 2012, pp. 887–890., doi:10.1016/j.fuel.2012.02.001.
[6] Das Neves, Marcos A, et al. “State of the Art and Future Trends of Bioethanol Production.” Global Science Books, 29 Apr. 2007.
[7] “Ethanol Biorefineries.” Ethanol Biorefineries - an Overview | ScienceDirect Topics, Elsevier, www.sciencedirect.com/topics/engineering/ethanol-biorefineries.
[8] Lipnizki, Frank. “Membrane Process Opportunities and Challenges in the Bioethanol Industry.” Desalination, vol. 250, no. 3, 2010, pp. 1067–1069., doi:10.1016/j.desal.2009.09.109.
[9] Zheng, Yi, et al. “Integrating Sugar Beet Pulp Storage, Hydrolysis and Fermentation for Fuel Ethanol Production.” Applied Energy, vol. 93, 2012, pp. 168–175., doi:10.1016/j.apenergy.2011.12.084.
[10] Moss, Angelique. “3 Emerging Bioethanol Businesses That Are Making Waves in the Market.” Medium, Small Business Forum, 27 Mar. 2018, smallbusinessforum.co/emerging-bioethanol-businesses-9b7c78a630ab.
[11] Karimi K., Chisti Y. “Future of bioethanol…” Biofuel Research Journal 5 (2015) 147-147
[12] Chen, Hongzhang, and Weihua Qiu. “Key Technologies for Bioethanol Production from Lignocellulose.” Biotechnology Advances, vol. 28, no. 5, 28 May 2010, pp. 556–562., doi:10.1016/j.biotechadv.2010.05.005.
[13] Tomas-Pejo, E, et al. “Realistic Approach to Full-Scale Bioethanol Production from Lignocellulose.” Journal of Scientific and Industrial Research, vol. 67, 22 Sept. 2008, pp. 874–884.
The content & opinions in this article are the author’s and do not necessarily represent the views of AltEnergyMag
Comments (0)
This post does not have any comments. Be the first to leave a comment below.
Featured Product
