In this paper, we look at the current state of value of solar (VOS) analysis and propose a more holistic methodology that can be consistently applied across various utility service areas.
Steven Fine, Ankit Saraf, Kiran Kumaraswamy, Alex Anich | ICF International
Executive Summary
As solar becomes an increasingly significant factor in the generation mix, ICF believes that utilities, investors, and markets are missing out on optimal strategies to price assets, lower costs, and mitigate risks, because they lack a consistent and accurate approach for determining the true value of solar. Current methodologies are all over the map, yielding different outputs based on different inputs, and different assumptions to those inputs. That is why we suggest here an updated, comprehensive methodology that could help all stakeholders expose the benefits and costs and make more informed—and ultimately more beneficial—investments.
This kind of approach will likely become even more important in the years to come. The amount of distributed photovoltaic (DPV) installed behind the meter specifically for residential customers grew 60 percent from 2012 to 2013. ICF forecasts DPV installations to continue to increase to over 27 GW on commercial and residential premises by 2018, representing over 1.8 million locations where DPV will be interconnected to the distribution grid. Utilities, regulators, and the broader solar industry will need to understand how to value this growing factor in the market.
In this paper, we look at the current state of value of solar (VOS) analysis and propose a more holistic methodology that can be consistently applied across various utility service areas. We recommend factors for inclusion and exclusion in the calculation and consider the most appropriate way to construct each variable, keeping in mind our base view that distributed resources need to be evaluated in the same light as other generating resources on the grid, not just a decrement to load.
We recommend the following methodological approaches on potential VOS components:
- Energy: avoided generated energy represents the most straight-forward calculation, and while the best way to quantify energy value would be at the margin, a more simplified interim approach may be considered.
- Avoided/Deferred Generation Capacity: there needs to be a realistic analysis of the correlation between customer and system peak as well as an analysis of the DPV’s generation profile to accurately assign a DPV system capacity credit. The same process should be applied to DPV projects as with central station renewables, with the added complication that penetration rates on distribution system feeders also need to be included.
- Avoided Transmission and Distribution (T&D) Losses and Capacity: For the T&D portion of the grid, DPV potentially represents both a benefit and a cost, and both of these impacts need to be included and separately assessed as part of a VOS analysis. This includes careful consideration of where DPV will be deployed versus the loads being served, as well as understanding the different feeder characteristics.
- Grid Support Services: based on the high amount of uncertainty on this variable, we believe that Grid Support Services should not be included in a VOS calculation for potential payment but should be reviewed for the potential to determine the mechanisms that may need to be in place to monetize any potential value when the appropriate technology exists.
- Environmental: as a generation resource on the grid, DPV should be evaluated in the same way as any other new resource, including central station renewables, in terms of environmental costs and benefits that it confers upon the system. The emissions (in this case CO2) values assigned on a $/ton basis should be similar to those used to evaluate other power generation resources.
- Financial: “financial” benefits such as a fuel price hedge, reservation of natural gas pipeline capacity, and/or a market price response are not typically accounted for in utility integrated resource planning (IRP) evaluations of new resources, including central station renewables, or in cost-benefit evaluations of EE measures, and should not be included in VOS analyses.
- Social: “social” values are not included in the construction of utility scale conventional or renewable projects and should not be part of VOS analyses.
- Security: when DPV is able to isolate from the grid or “island,” it can provide reliability to the owner of the facility. This value, however, does not accrue to society or even the local grid. Security benefits should therefore be explored to value resiliency at specific critical locations, however, they should not yet be included in the VOS calculation until a set and agreed-upon methodology for resiliency is developed.
- Frequency to Update VOS: the utility should update the VOST on a locational basis for new installations yearly to address the changing amount of solar or energy use in different areas.
The result of our approach is a general roadmap for achieving a better consensus VOS, though calculations for individual entities would require an analysis tailored to the individual circumstances of their geography, energy market, and physical grid infrastructure.
This new VOS calculation could be an input in calculating the retail credit net energy metering (NEM) subsidy under a Value of Solar Tariff (VOST), and can also help to guide larger investment and market decisions for utilities, regulators, and the broader solar industry, better aligning costs and benefits and mitigating the risks that the rise of solar will bring to the market.
The Value of Solar
Value of Solar (VOS) Analysis to Date
Numerous studies have been conducted on VOS, but these have been highly variable in generating estimated value due to the different approaches used to calculate benefits and costs. Despite these variations in values, VOS studies do commonly agree upon the broad categories involved, although not consistently. Figure 1 illustrates the broad categories commonly used in VOS studies extrapolated from the Rocky Mountain Institute’s (RMI) recent 2013 meta-study “A Review of Solar PV Benefit and Cost Studies.” These categories cover most of the tangible and intangible benefits associated with distributed solar.
Figure 1. Typical Solar PV Benefit and Cost Categories1
A recent ICF study reviewed five of the VOS studies included in RMI’s analysis and found them lacking in consistency, key value and cost components, and transparency. Figure 2 below shows the wide range of VOS estimates in these studies—from $50/MWh to over $250/MWh - as well as the benefit and cost estimates by category.
Most of these recent VOS studies also do not capture and consistently value the true cost and benefit of integrating solar DG into the system. Specifically, these studies often overlook the need to maintain reliable electric service to the end-use customer, as well as the fact that the grid is the enabling mechanism for DPV.
For the majority of the public VOS analysis reviewed here, benefits consistently manifested themselves in avoided energy and capacity purchases as well as transmission and distribution (T&D) impacts. Categories such as financial risk, grid support services and reduced security risk were valued in some, but not in other studies. The difference in estimations and exclusion of value components from multiple VOS studies further emphasizes the need for more consistent methods in valuing solar.
Figure 2. Recent VOS Studies2: Range of VoS Cost and Benefit Price Estimates
Incompatible Peak LoadsThe vast majority of customers who install solar DG will still rely on the grid to absorb the excess generation from solar PV in the middle of the day when the solar system is producing its peak power (and household consumption is typically low). The grid is also needed to reliably and consistently deliver electricity in the evenings when solar generation is non-existent (and when household electricity consumption is typically high). Figure 3 from a residential system in Southern California highlights this challenge.
Figure 3. Illustrative DPV Generation Profile vs. Customer Load Storage is changing this, but very slowly, and it is impractical to expect millions of solar PV deployments to install storage and depart from the grid in the near to mid-term. Emphasis therefore must be placed on the cost of maintaining reliable electric service to all end-use customers. While solar brings incremental value to the system when locational generation is needed, the maintenance of the grid also brings significant value to the end-use customer. |
Our Proposed Approach to VOS
- An analysis of some of the “traditional” components of VOS analysis;
- Treatment of DPV as a locational generation resource and not as a decrement to load.
-
Energy. Avoided generated energy represents the most straight-forward calculation across existing VOS studies. The premise is that energy generated from solar DG installation displaces generation from the grid. Current VOS studies generally assume that solar DG displaces gas-fired combined cycle resources over the daily generation profile of the solar resource. While a more accurate estimate could be obtained using hourly dispatch modeling to determine the marginal resource in each hour, this is a reasonable assumption given the relatively low levels of DPV penetration on the grid. As DPV penetration rates increase, however, a more rigorous analysis involving dispatch modeling may be required in areas where other resources might be displaced.
Overall, while some of the studies we examined identified high avoided energy costs due largely to inflated natural gas price assumptions, there is generally little disagreement about avoided energy costs for VOS.
Utilities should ideally develop appropriate time of delivery values for the energy component of supply and consider publishing time of use prices that convert the marginal resources into a time differentiated tariff.
- Avoided Generation Capacity. Unlike calculating displaced energy from DPV, valuing avoided capacity is both more complicated and often overstated in many existing VOS analyses. Avoided generation capacity is the amount of capacity (MW) that can be deferred due to the DPV installation, and its value hinges on two central factors.
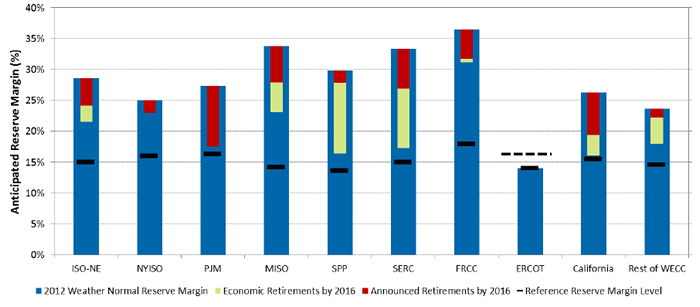
- Avoided Transmission and Distribution (T&D) Losses and Capacity. The avoided T&D losses and capacity component of the VOS refers to two distinct issues. One is the T&D infrastructure that can be avoided or deferred due to the reduced need to supply a customer that consumes power generated on his or her rooftop. The second is the T&D losses that can be avoided by consuming power adjacent to the point of consumption, as opposed to needing to transmit it long distances from a central generating facility.
- If the T&D deferral should be valued on an average versus a marginal basis
- If energy losses should be evaluated, and if so, whether it should be done on a peak versus all-hours basis
- Should transmission and distribution be treated as two distinct systems, or as effectively one
Example from Los Angeles County
ICF performed an analysis of varying degrees of DPV concentration and penetration levels on a network of distribution feeders in Los Angeles County which demonstrated the importance of understanding the correlation between DPV generation, feeder load and the resulting impact on voltage violations (See Figure 5).
Figure 5. Time Series Analysis (Typical Summer Day) with Solar PV at High Load Locations1
![]() |
Grid Support Services.
- Environmental: The deployment of DPV has environmental value as an emissions-free source of generation for the amount of fossil fuel-fired generation it displaces from the grid. Since many VOS analyses assume that the displaced generation is coming from combined cycle gas generation, the avoided emissions are assigned a similar value – generally around 800 to 1000 lb. CO2/MWh, depending on the efficiency of the combined cycle unit. Going forward, as central station renewables and DPV penetration on the grid increases, more detailed analysis should be performed to determine the emissions characteristics of the marginal unit being displaced. This unit could be natural gas, coal, wind, hydro or even other renewables.
RMI Categories Not Included in ICF VOS Methodology |
---|
We believe that the below categories listed in the RMI metadata analysis should not be part of a VOS estimate. Below is a description of these categories and our reasoning for exclusion.
Central station and large generating assets should coordinate with one another through an RTO and distribution utility in order to provide optimal grid resiliency. In contrast, it has been suggested that DPV should provide a degree of resiliency to the grid by diversifying the generating assets in terms of supply and location; however, DPVs have neither the scale nor the communication infrastructure to coordinate and provide resiliency value. DPV’s impact on reliability and resiliency is evolving as utility communication to these assets improves, and as strategies to recover costs or monetize the value of DPV to distribution feeders, community and utilities are developed. These values will be realized and likely maximized by the utility in determining appropriate locations and services.
Individually-owned systems that can be islanded have a value, but the value accrues to the owner of the system, and therefore it should not be part of a VOS calculation. If DPVs are able to be controlled and managed by the electric utility—along with other grid stabilizing equipment on a feeder basis similar to a micro grid—then there is locational value. This should be estimated and included in a VOS analysis as a resource that is rarely used but, when it is, brings significant value.
|
Frequency to Update VOS:
Conclusion
References
1 Derived from RMI “A Review of Solar PV Benefit and Cost Studies” by ICF. www.rmi.org/Knowledge-Center%2FLibrary%2F2013-13_eLabDERCostValue
2 List of Studies Considered: CPRTX - The Value of Distributed Solar Electric Generation to San Antonio. Clean Power Research, 2013; CPRNP - The Value of Distributed Solar Electric Generation to NJ and PA. Clean Power Research, 2012; SAICAZ - 2013 Updated Solar PV Value Report (for Arizona). SAIC, 2013; CBEAZ - Evaluating the Benefits and Costs of Net Energy Metering in CA. Crossborder Energy, 2013; CBECA - Evaluating the Benefits and Costs of Net Energy Metering in AZ. Crossborder Energy, 2013.
3 See the much publicized “duck curve” at www.caiso.com/documents/flexibleresourceshelprenewables_fastfacts.pdf
4 See http://energy.sandia.gov/wp/wp-content/gallery/uploads/SAND2012-1365-v2.pdf for a detailed explanation on the causes of feeder unintentional islanding due to solar PV penetrations
About the Authors
About ICF International
The content & opinions in this article are the author’s and do not necessarily represent the views of AltEnergyMag
Comments (0)
This post does not have any comments. Be the first to leave a comment below.
Featured Product
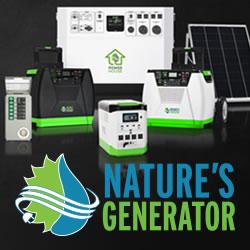