Compared to other mechanisms that convert chemical energy into electricity, the fuel cell, specifically the solid oxide or ceramic fuel cell, is the most efficient.
Fuel Cell Technology for the Future
Len Calderone for | AltEnergyMag
It has been a dream to be able to achieve the benefits of clean electric power without pollution producing engines or heavy batteries. Solar and wind technology has been in the forefront of this search, but these technologies have inherent problems. If the wind doesn’t blow, the windmills don’t turn; and if the clouds cover the sun, solar power is limited. These technologies are also not portable in some areas of use, such as tractor-trailers.
Clean energy is now the big topic of discussion and fuel cell technology is growing. A fuel cell is an electrochemical mechanism that converts the chemical energy in fuels, using sources, such as hydrogen, methane, butane into electrical energy through the reaction of oxygen and hydrogen. Energy can be obtained by this reaction. Fuel cells are simple devices, containing no moving parts and only four functional component elements, which are the cathode, electrolyte, anode, and interconnect.
Inserted between an anode and a cathode is an ion-conducting material called an electrolyte. Fuel flows in one side, over the anode, while an oxidant flows into the other side, over the cathode. The fuel and the oxidant react and the sparks that fly from that encounter are actual electrons, which flow into the fuel cell's circuit, resulting in electricity.
A fuel cell is a power plant and not a battery. Once it converts fuel to energy, it sends that energy to work, requiring some peripheral way to physically store the fuel ingredients, needing some way to capture produced electricity, such as a battery.
There are several types of fuel cells, the most well known is the “hydrogen fuel cell,” used in cars. These fuel cells use hydrogen as a fuel and oxygen as an oxidant.
Bloom Energy's Energy servers are of the solid oxide variety of fuel cell. There's two ways to make a SOFC (solid oxide fuel cell). One design is like the one pictured above, a tubular design. Bloom Energy uses a planar design, which allows them to be stacked into very neat boxes.
As can be seen on the illustration above, the two components required to induce the electro-chemical reaction is methane (CH4) and oxygen (O2). Methane is the main component in natural gas, which is the fuel source that runs most Bloom units today. The fuel cells can also run on biogas, which can be produced by animal waste, landfill waste, algae or any other organic matter.
Solid oxide fuel cells are made from solid state materials, similar to ceramic material. Bloom Energy claims their fuel cells are made out of "sand," baked into ceramic squares. The exact material is proprietary, including the black and green inks that coat the plates.
A SOFC can reduce the carbon footprint by 40% to100% compared to using electricity from the existing power grid. As no combustion is involved, combustion related climate gases are not released into the atmosphere.
One of selling points of SOFCs is freedom from the utility grid. This means that companies that invest in this technology are less reliant on power failures and increasing electricity prices. Eventually, there could be a fuel cell in every home, replacing the power grid.
Besides being attractive energy sources, SOFCs are clean, reliable, and almost entirely nonpolluting. They are also vibration-free and quiet.
The cathode must be porous in order to allow oxygen molecules to reach the electrolyte interface. In some designs, the cathode contributes over 90% of the cell's weight and provides structural support for the cell. Today the most commonly used cathode material is lanthanum manganite, which is an oxide ceramic material. This material is commonly used in manufacturing commercially produced SOFCs because it has a high electrical conductivity at higher temperatures, and its thermal expansion coefficient is well matched with yttria-stabilized zirconia, a common material for SOFC electrolytes.
Once the molecular oxygen has been converted to oxygen ions it must travel through the electrolyte to the fuel side of the cell. The electrolyte must possess a high ionic conductivity and no electrical conductivity for this to happen. It must be fully dense to prevent short circuiting of reacting gases through it and it should also be as thin as possible to minimize resistive losses in the cell; plus it must be chemically, thermally, and structurally constant across a wide temperature range.
The anode must meet the same requirements as the cathode for electrical conductivity, thermal expansion and porosity. The reducing conditions combined with electrical conductivity requirements make metals attractive candidate materials. Development has centered on nickel because it is affordable and abundant.
An internal combustion engine relies on several cylinders to provide enough power to be functional, and fuel cells must be used in combination in order to generate enough voltage and current. An interconnect functions as the electrical contact to the cathode while protecting it from the reducing atmosphere of the anode
CFY (chromium (Cr), iron (Fe) and yttrium (Y) interconnect by PLANSEE SE
The interconnects must meet the most stringent requirements of all of the components, because of high temperatures and severe environments. They must have 100% electrical conductivity, have no porosity, be compatible with thermal expansion, and be inert with respect to the other components. It will be exposed simultaneously to the reducing environment of the anode and the oxidizing atmosphere of the cathode.
The Fraunhofer Institute for Ceramic Technologies IKTS (Germany) designed a hot box with eight fuel cell stacks in series. The initial test of the newly developed hot box was completed successfully in March 2012. During the test, the stack module achieved a power output of 5.5 kW. Following a further test using simulated natural gas reformate, the stack module will be installed in a specially developed system environment at AVL List GmbH, where it will be tested. AVL is the world's largest privately owned company for development, simulation and testing technology of powertrains (powerplants).
Delphi Automotive Systems is developing an SOFC that will power auxiliary units in automobiles and tractor-trailers. A high-temperature SOFC will generate all of the needed electricity to allow the engine to be smaller and more efficient. The SOFC would run on the same gasoline or diesel as the engine and would keep the air conditioning unit and other necessary electrical systems running while the engine shuts off when not needed.
Lilliputian Systems, Inc. is looking to launch its pocket size fuel cell charger, the USB Mobile Power System, a revolutionary portable system for powering and charging virtually all mobile phones and countless other consumer electronic devices. The device can provide between 10 and 14 charges for smart phones. It’s about the size of a deck of cards and is fueled by replaceable cartridges filled with butane, or lighter fluid.
The vulnerability of fuel supply lines for the military is notorious. Diesel generators are the primary solutions used to support FOBs (forward operating base), and they are dirty, noisy and inefficient. The cost of delivering diesel fuel to FOBs ranges from $40 per gallon to $400 per gallon. Lockheed is developing fuel cell technology with the support of the Office of Naval Research, which will be designed to integrate with solar photovoltaic panels, which will reduce the amount of fuel needed to support forward operating military bases.
Fuel cells are being steered toward use in power and heat generation for homes and businesses, as well as auxiliary power units for electrical systems in vehicles. SOFC also can be linked with a gas turbine, in which the hot, high pressure exhaust of the fuel cell can be used to spin the turbine, generating a second source of electricity.
Compared to other mechanisms that convert chemical energy into electricity, the fuel cell, specifically the solid oxide or ceramic fuel cell, is the most efficient.
For further information:
1. http://www.benwiens.com/energy4.html
2. http://www.ecw.org/prod/207-R.pdf
3. http://electrochem.cwru.edu/encycl/art-f02-sofc.htm
4. http://addis.caltech.edu/publications/Fuel%20Cell%20Mats%20%26%20Comps%20corr.pdf
5. http://www.ece.uic.edu/~mazumder/8.pdf
About LenLen contributes articles to several technology eMagazines, and has written a DIY wedding photography book, which is being published in 2013. He also writes a Op-Ed column for a local newspaper. This article contains statements of personal opinion and comments made in good faith in the interest of the public. You should confirm all statements with the manufacturer to verify the correctness of the statements. |
![]() |
The content & opinions in this article are the author’s and do not necessarily represent the views of AltEnergyMag
Comments (1)
Featured Product
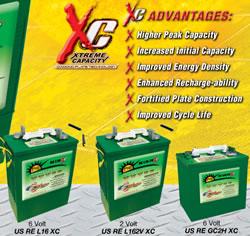